Dynamics and Physics
Understanding Tropical Cyclone Structure and Intensity
SCROLL TO LEARN MORE
Who We Are
The destructive potential of a hurricane is governed by its interaction with the environment and physical processes internal to the storm. Researchers at AOML use a variety of tools to better understand how phenomena from the larger environmental scale down to the cloud and turbulent scale interact (multi-scale interactions) to produce such changes.
We also work closely with scientists from other disciplines to improve the tools available and to apply a new understanding in a way that benefits prediction and improves forecasts.
Objective
Our Objective is to improve our understanding of tropical cyclone structure and intensity change through the application of fundamental physical principles.
Read More News
Components of the Multi-Scale Interaction
Storm Environment
A hurricane develops within an atmosphere characterized by evolving larger-scale distributions of moisture and wind, and over an ocean with a more slowly-evolving distribution of temperature. The surrounding atmosphere and ocean below a hurricane is called
the storm environment. Because the storm environment interacts with the hurricane, it is important both to characterize and understand it.
Storm-Environment Interactions
The interaction between a tropical cyclone (whether a hurricane, tropical storm, tropical depression, or a pre-depression) and the environment it encounters plays a critical role in determining whether the storm structure and intensity will change.
Internal Storm Processes
These are physical processes that can operate independently of the storm’s environment, or “internally.” Among other impacts, they are often associated with dramatic short-term changes in storm size and intensity, and are responsible for intense localized winds.
Building a Weather-Ready Nation.
Understanding Hurricane Formation.
Read “Precipitation Processes and Vortex Alignment during the Intensification of a Weak Tropical Cyclone in Moderate Vertical Shear.”
Understanding the formation of a hurricane is critical to preparing coastal communities before severe weather threatens our coasts. Our scientists are studying this question by looking at how these weather patterns change and mature in their environment from small to large scales. Read the latest research below.
Vortex Alignment
Storms struggle to intensify when their circulations are not aligned between the lower and upper levels of the atmosphere, which often happens when they encounter vertical wind shear. AOML hurricane scientists investigate how these storms can become aligned and intensify, even in the presence of this hostile wind environment.
Precipitation Processes
The structure of precipitation (rainfall) and how it’s distributed around the storm can play an important role in determining whether or not the storm will intensify. Scientists at AOML investigate how the structure and distribution of precipitation changes in relation to the storm environment to allow for intensification.
Planetary Boundary Layer Recovery
Precipitation can bring cool, dry air to the boundary layer, effectively limiting the development of strong thunderstorms in the storm circulation. Scientists at AOML study how the boundary layer can recover in the presence of vertical wind shear to allow for new development of thunderstorms and storm intensification.
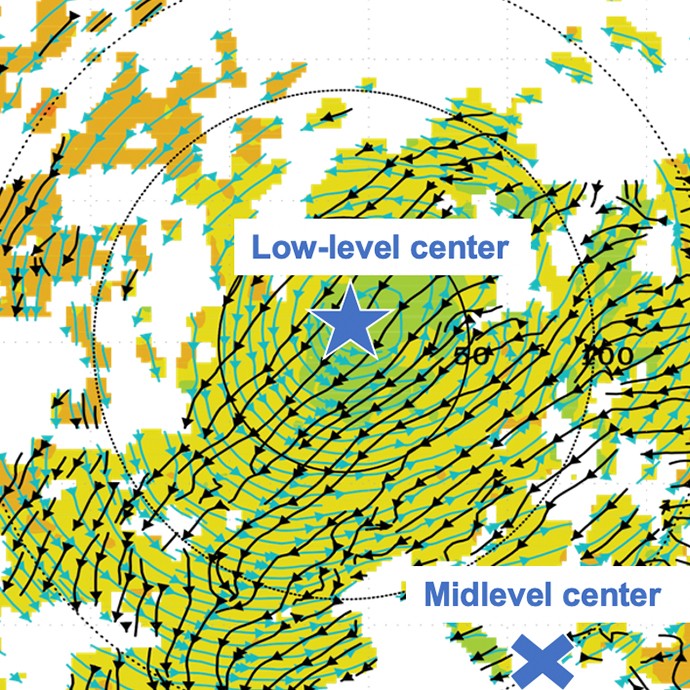
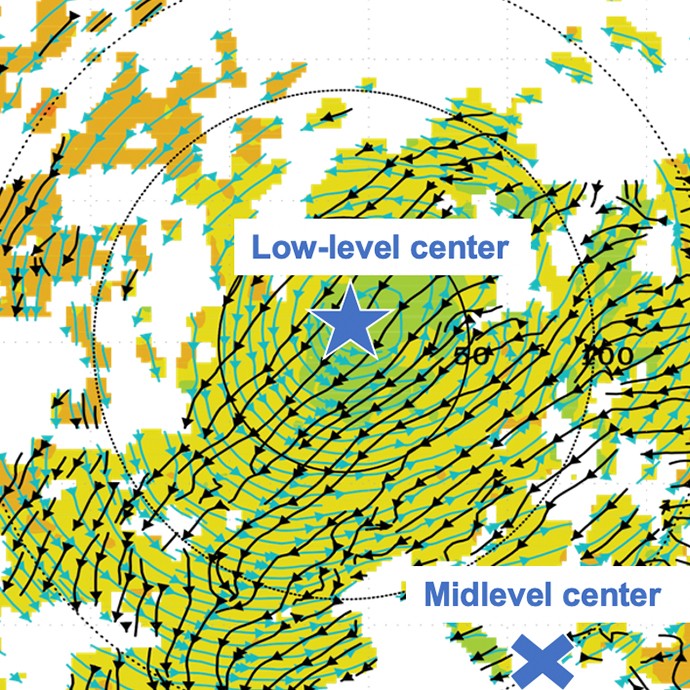
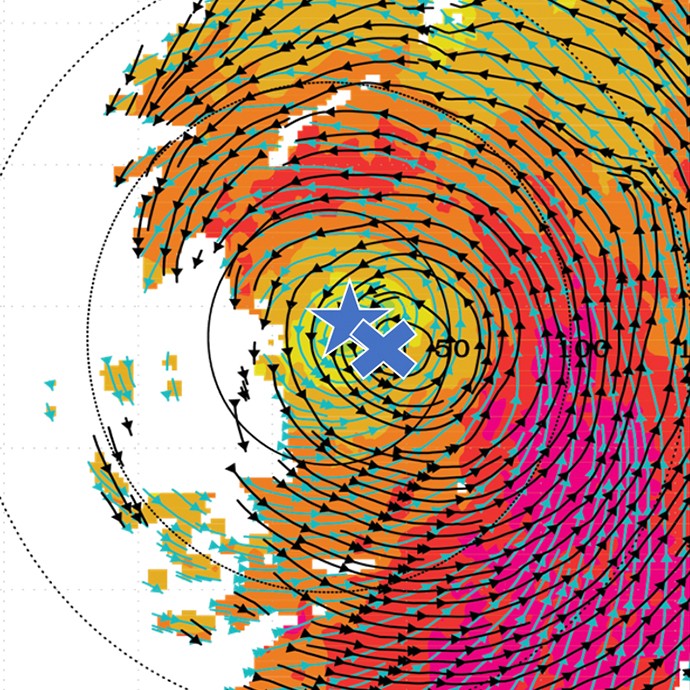
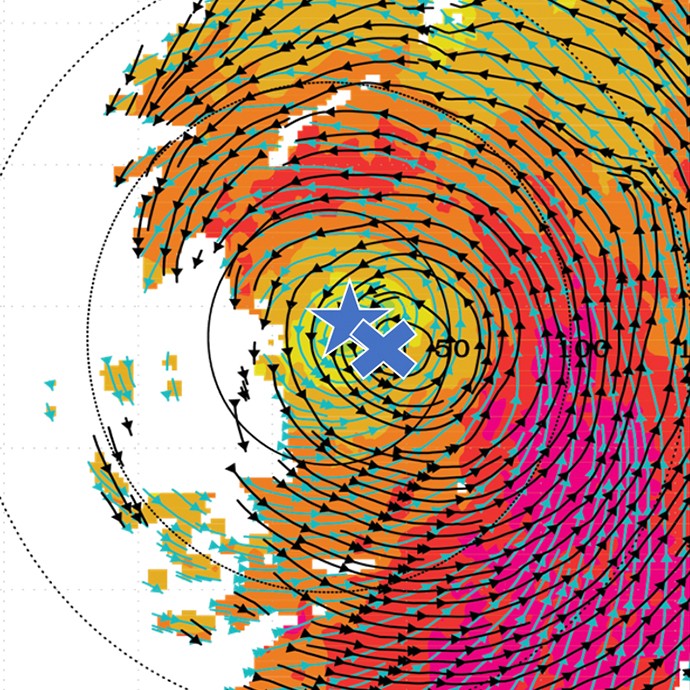
Slide to look at aligned v. unaligned vortex
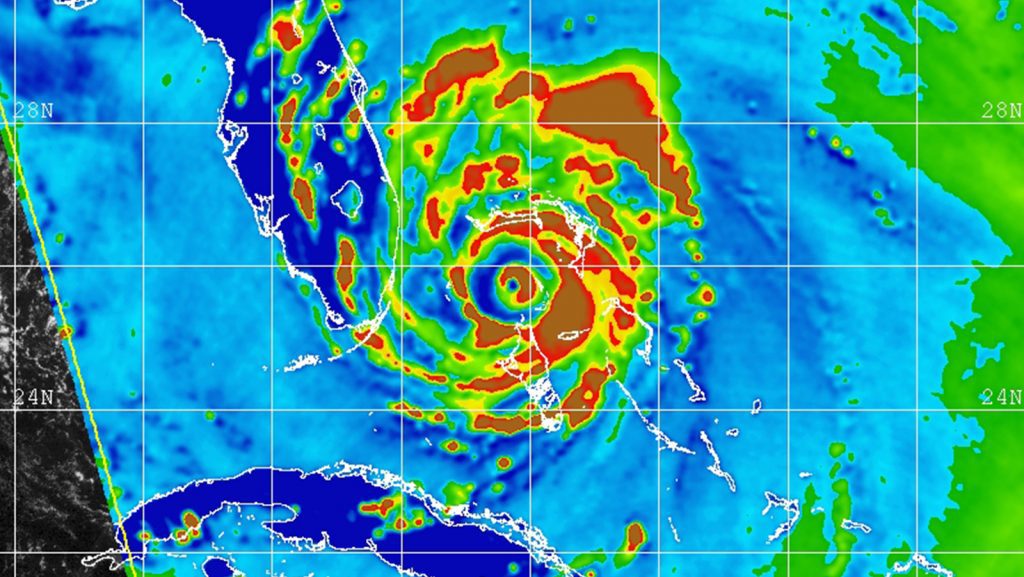
Secondary Eyewall Formation
Intense hurricanes often develop so-called secondary eyewalls outside of the initial primary eyewall. AOML hurricane scientists investigate how these secondary eyewalls form and their impact on hurricane intensity.
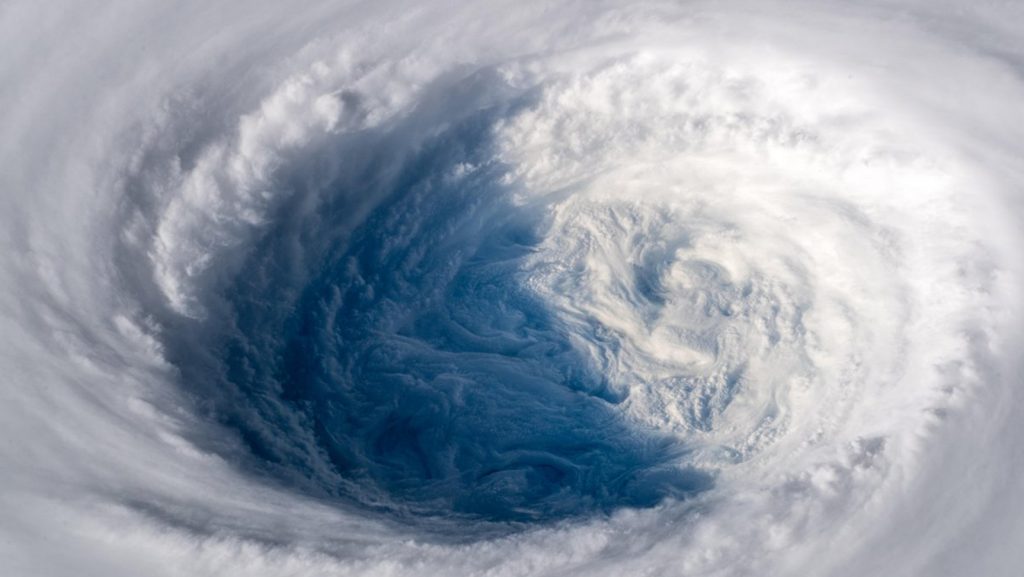
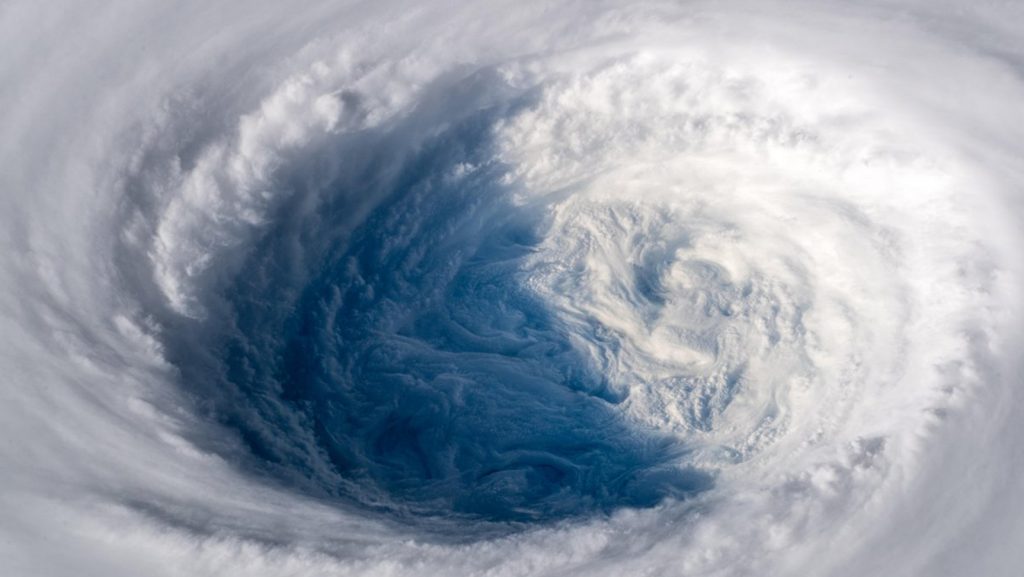
Eyewall Mixing
An otherwise circular eyewall can transition into a dynamic mixture of spiral bands and intense localized swirls. Hurricane scientists at AOML investigate how these smaller-scale structures impact hurricane intensification.
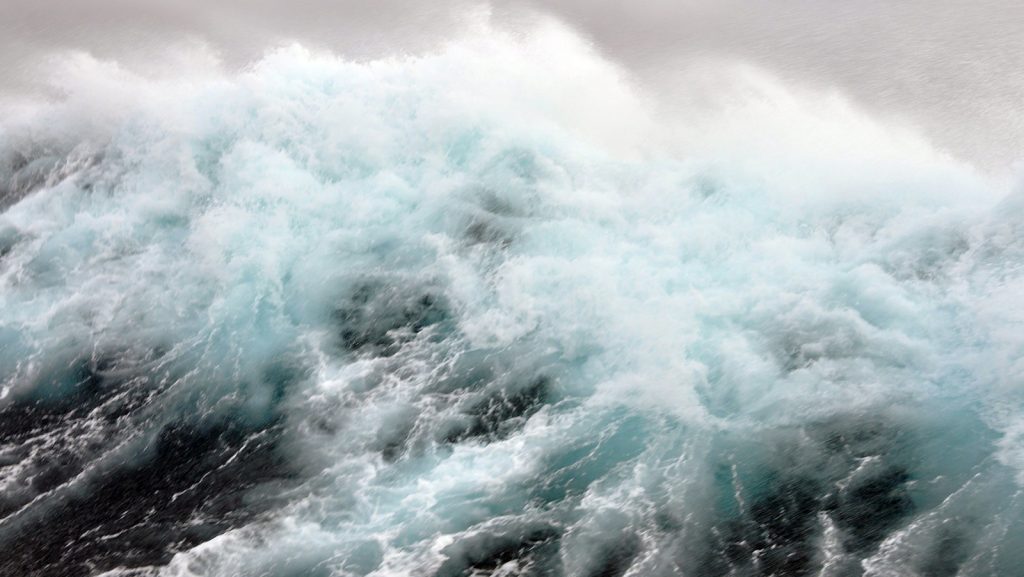
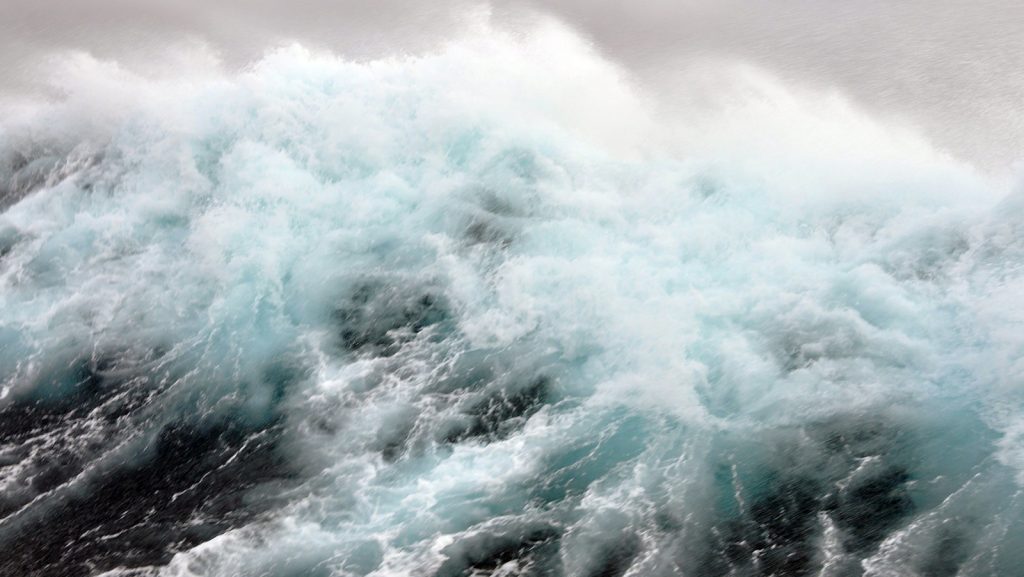
Planetary Boundary Layer Dynamics
The layer of the hurricane flow closest to the ocean surface is characterized by turbulent motion. AOML scientists study how the effects of turbulence can be represented in models that are unable to simulate the turbulent motions. They also study how the turbulence contributes to hurricane intensification.
Hurricane Field Program
We Drive Innovative Science.
Experimental Design and the Hurricane Field Program.
Addressing research questions about the dynamics and physics of tropical cyclones is a major factor in how in-flight observations are taken. Because we have a direct line to observations from the Hurricane Field Program, we can capitalize on using these data for cutting-edge hurricane genesis and intensification research.
Read the experimental design plans for the Hurricane Field Program to learn more.
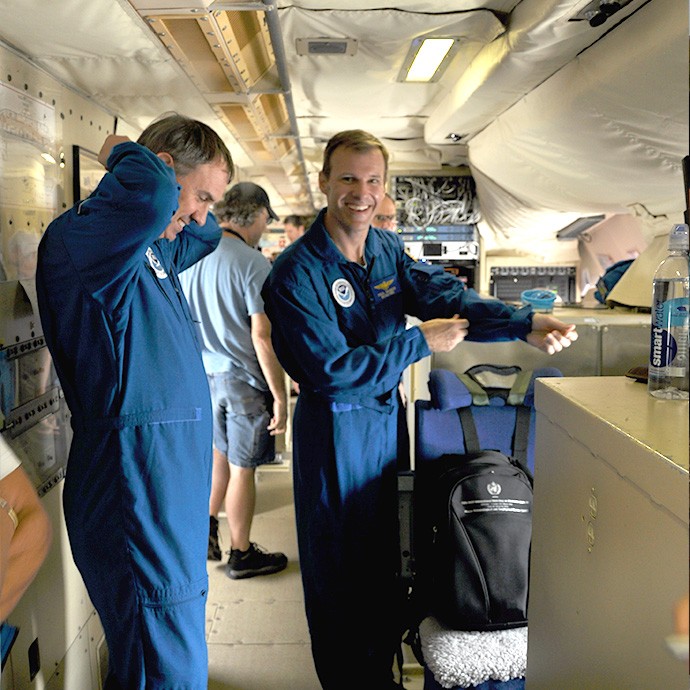
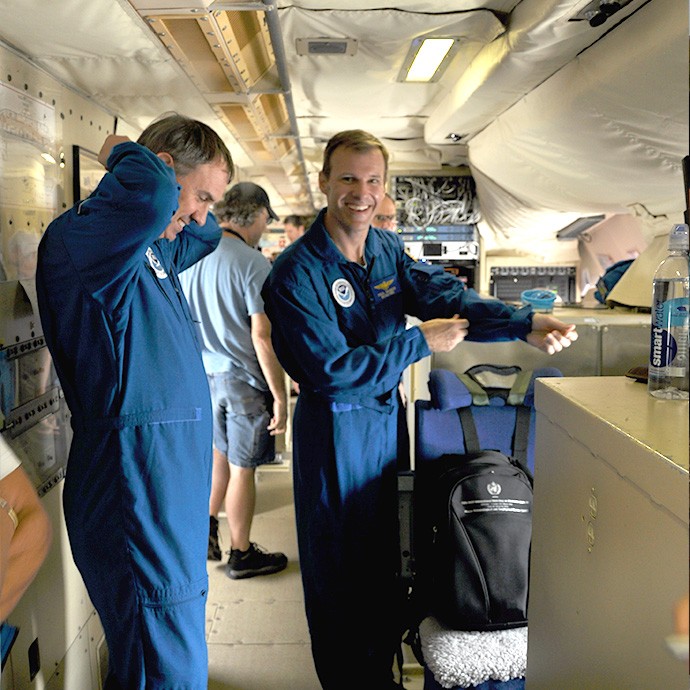
Publications & References
2020
Cione, J.J., G. Bryan, R. Dobosy, J. Zhang, G. de Boer, A.Aksoy, J. Wadler, E.Kalina, B. Dahl, K. Ryan, J. Neuhaus, E. Dumas, F. Marks, A. Farber, T. Hock and X. Chen 2020: Eye of the Storm: Observing Hurricanes with a Small Unmanned Aircraft System. Bull. Amer. Meteor. Soc. https://doi.org/10.1175/BAMS-D-19-0169.1 Fischer, M. S., R. F. Rogers, and P. D. Reasor, 2020: The Rapid Intensification and Eyewall Replacement Cycles of Hurricane Irma (2017). Mon. Wea. Rev., 148, 981–1004, https://doi.org/10.
1175/MWR-D-19-0185.1. Rogers, R. F., P. D. Reasor, J. A. Zawislak, and L. T. Nguyen, 2020: Precipitation Processes and Vortex Alignment during the Intensification of a Weak Tropical Cyclone in Moderate Vertical Shear. Mon. Wea. Rev., 148, 1899–1929, https://doi.org/10.1175/MWR-D-19-0315.1. 2019
Ahern, K., M.A. Bourassa, R.E. Hart, J.A. Zhang, and R.F. Rogers, 2019: Observed Kinematic and Thermodynamic Structure in the Hurricane Boundary Layer during Intensity Change. Mon. Wea. Rev., 147, 2765–2785, https://doi.org/10.1175/MWR-D-18-0380.1.Alvey III, G. R., E. Zipser, J. Zawislak, 2019: How does Hurricane Edouard (2014) evolve toward symmetry before intensification? A high-resolution ensemble study. J. Atmos. Sci., in press.
Chen, X., J. A. Zhang, and F. D. Marks, 2019: A thermodynamic pathway leading to rapid intensification of tropical cyclones in shear. Geophys. Res. Lett., 46, 9241-9251, https://doi.org/10.1029/2019GL083667
Chen X., J. A. Zhang, F. D. Marks, R. F. Rogers, and J. J. Cione, 2019: Precipitation Symmetrization and Rapid Intensification of Tropical Cyclones under Shear: J. Atmos. Sci., in review.
Dunion, J.P., C.D. Thorncroft, and D.S. Nolan. 2019: Tropical cyclone diurnal cycle signals in a hurricane nature run. Mon. Wea. Rev., 147, 363-388, https://doi.org/10.1175/MWR-D-18-0130.1.
Guimond, S.R., P.D. Reasor, G.M. Heymsfield, and M. McLinden, 2019: The Dynamics of Vortex Rossby Waves and Secondary Eyewall Development in Hurricane Matthew (2016): New Insights from Radar Measurements. J. Atmos. Sci., in review.
Martinez, J., M.M. Bell, R.F. Rogers, and J.D. Doyle, 2019: Axisymmetric potential vorticity evolution of Hurricane Patricia (2015), J. Atmos. Sci., 76, 2043–2063, https://doi.org/10.1175/JAS-D-18-0373.1.
Molinari, J., J.A. Zhang, R.F. Rogers, and D. Vollaro, 2019: Repeated Eyewall Replacement Cycles in Hurricane Frances (2004). Mon. Wea. Rev., 0, https://doi.org/10.1175/MWR-D-18-0345.1.
Nguyen, L.T., R.F. Rogers, J. Zawislak, and J.A. Zhang, 2019: Assessing the Influence of Convective Downdrafts and Surface Enthalpy Fluxes on Tropical Cyclone Intensity Change in Moderate Vertical Wind Shear. Mon. Wea. Rev., 0, https://doi.org/10.1175/MWR-D-18-0461.1.
Wadler, J., R.F. Rogers, and P.D. Reasor, 2018a: The relationship between spatial variations in the structure of convective bursts and tropical cyclone intensification as determined by airborne Doppler radar. Mon. Wea. Rev., 146, 761–780. https://doi.org/10.1175/MWR-D-17-0213.1.
Zhang, J.A. and R.F. Rogers, 2019: Effects of parameterized boundary layer structure on hurricane rapid intensification in shear. Mon. Wea. Rev., 147, 853-871, https://doi.org/10.1175/MWR-D-18-0010.1.
2018
Bowers, G. S., Smith, D. M., Kelley, N. A., Martinez‐McKinney, G. F., Cummer, S. A., Dwyer, J. R., et al. ( 2018). A terrestrial gamma‐ray flash inside the eyewall of Hurricane Patricia. Journal of Geophysical Research: Atmospheres, 123, 4977– 4987. https://doi.org/10.1029/2017JD027771Didlake, A.C., Paul D. Reasor, and R.F. Rogers, W.-C. Lee, 2018: Dynamics of the transition from spiral rainbands to a secondary eyewall in Hurricane Earl (2010). J. Atmos. Sci., 75, 2909–2929, https://doi.org/10.1175/JAS-D-17-0348.1.
Dougherty, E.M., J. Molinari, R.F. Rogers, J.A. Zhang, and J.P. Kossin, 2018: Hurricane Bonnie (1998): Maintaining Intensity during High Vertical Wind Shear and an Eyewall Replacement Cycle. Mon. Wea. Rev., 146, 3383–3399, https://doi.org/10.1175/MWR-D-18-0030.1
Guimond, S. R. J. A. Zhang, J. Sapp, and S. J. Frasier, 2018: Coherent turbulence in the boundary layer of Hurricane Rita (2005) during an eyewall replacement cycle. J. Atmos. Sci., 75, 3071–3093.
Leighton, H., S. Gopalakrishnan, J.A. Zhang, R.F. Rogers, Z. Zhang, and V. Tallapragada, 2018: Azimuthal Distribution of Deep Convection, Environmental Factors, and Tropical Cyclone Rapid Intensification: A Perspective from HWRF Ensemble Forecasts of Hurricane Edouard (2014). J. Atmos. Sci., 75, 275–295, https://doi.org/10.1175/JAS-D-17-0171.1
Wadler, J.B., J.A. Zhang, B. Jaimes, and L.K. Shay, 2018b: Downdrafts and the Evolution of Boundary Layer Thermodynamics in Hurricane Earl (2010) before and during Rapid Intensification. Mon. Wea. Rev., 146, 3545–3565.
2017
Didlake, A.C., G.M. Heymsfield, P.D. Reasor, and S.R. Guimond, 2017: Concentric Eyewall Asymmetries in Hurricane Gonzalo (2014) Observed by Airborne Radar. Mon. Wea. Rev., 145, 729–749, https://doi.org/10.1175/MWR-D-16-0175.1Hazelton, A.T., R.F. Rogers, and R.E. Hart, 2017: Analyzing simulated convective bursts in two Atlantic hurricanes. Part I: Convective burst formation and development. Mon. Wea. Rev., 145(8), 3073-3094, doi: 10.1175/MWR-D-16-0267.1.
Hazelton, A.T., R.E. Hart, and R.F. Rogers, 2017: Analyzing simulated convective bursts in two Atlantic hurricanes. Part II: Intensity change due to convective bursts. Mon. Wea. Rev., 145(8), 3095-3117, doi: 10.1175/MWR-D-16-0268.1.
Kalina, E.A., S. Matrosov, J. Cione, F. Marks, J. Vivekanandan, R. Black, J. Hubbert, M. Bell, D. Kingsmill, and A. White 2017: The Ice Water Paths of Small and Large Ice Species in Hurricanes Arthur (2014) and Irene (2011). J. Appl. Meteorol. DOI: http://dx.doi.org/10.1175/JAMC-D-16-0300.1
Martinez, J., M.M. Bell, J.L. Vigh, and R.F. Rogers, 2017: Examining Tropical Cyclone Structure and Intensification with the FLIGHT+ Dataset from 1999 to 2012. Mon. Wea. Rev., 145, 4401–4421, https://doi.org/10.1175/MWR-D-17-0011.1.
Nguyen, L.T., R.F. Rogers, and P.D. Reasor, 2017: Thermodynamic and Kinematic Influences on Precipitation Symmetry in Sheared Tropical Cyclones: Bertha and Cristobal (2014). Mon. Wea. Rev., 145, 4423–4446, https://doi.org/10.1175/MWR-D-17-0073.1.
Smith, R. K., J. A. Zhang and M. T. Montgomery, 2017: The dynamics of intensification in a Hurricane Weather and Research Forecasting simulation of Hurricane Earl (2010). Q. J. R. Meteorol. Soc., 143, 297-308.
Zhang, J.A., R.F. Rogers, and V. Tallapragada, 2017: Impact of Parameterized Boundary Layer Structure on Tropical Cyclone Rapid Intensification Forecasts in HWRF. Mon. Wea. Rev., 145, 1413–1426, doi: 10.1175/MWR-D-16-0129.1.
2016
Abarca, S.F., M.T. Montgomery, S.A. Braun, and J.P. Dunion, 2016: On the secondary eyewall formation of Hurricane Edouard (2016), Mon. Wea. Rev. 144, 3321-3331, https://doi.org/10.1175/MWR-D-15-0421.1.Guimond, S.R., G.M. Heymsfield, P.D. Reasor, and A.C. Didlake, 2016: The Rapid Intensification of Hurricane Karl (2010): New Remote Sensing Observations of Convective Bursts from the Global Hawk Platform. J. Atmos. Sci., 73, 3617–3639, https://doi.org/10.1175/JAS-D-16-0026.1
Rogers, R.F., J.A. Zhang, J. Zawislak, H. Jiang, G.R. Alvey III, E.J. Zipser, and S.N. Stevenson, 2016: Observations of the structure and evolution of Hurricane Edouard (2014) during intensity change. Part II: Kinematic structure and the distribution of deep convection. Mon. Wea. Rev., 144, 3355-3376.
Zawislak, J., H. Jiang, G.R. Alvey III, E.J. Zipser, R.F. Rogers, J.A. Zhang, and S.N. Stevenson, 2016: Observations of the structure and evolution of Hurricane Edouard (2014) during intensity change. Part I: Relationship between the thermodynamic structure and precipitation. Mon. Wea. Rev., 144, 3333-3354.
2015
Cione, J.J. 2015: The relative roles of the ocean and atmosphere as revealed by buoy air-sea observations in hurricanes. Mon. Wea. Rev. doi: 10.1175/MWR-D-13-00380.1Hazelton, A., R.F. Rogers, and R.Hart, 2015: Shear-Relative Asymmetries in Tropical Cyclone Eyewall Slope. Mon. Wea. Rev., 143, 883-903.
Reasor, P.D. and M.T. Montgomery, 2015: Evaluation of a Heuristic Model for Tropical Cyclone Resilience. J. Atmos. Sci., 72, 1765–1782, https://doi.org/10.1175/JAS-D-14-0318.1
Rogers, R.F., P.D. Reasor, and J.A. Zhang, 2015: Multiscale structure and evolution of Hurricane Earl (2010) during rapid intensification. Mon. Wea. Rev., 143, 536-562.
Susca-Lopata, G., J. Zawislak, E.J. Zipser, and R.F. Rogers, 2015: The role of observed environmental conditions and precipitation evolution in the rapid intensification of Hurricane Earl (2010). Mon. Wea. Rev., 143, 2207-2223.
Zhang, J. A., and F. D. Marks, 2015: Effects of horizontal diffusion on tropical cyclone intensity change and structure in idealized three-dimensional numerical simulations, Mon. Wea. Rev., 143, 10: 3981-3995.
Zhang, J. A., D. S. Nolan, R. F. Rogers, and V. Tallapragada, 2015: Evaluating the impact of improvements in the boundary layer parameterization on hurricane intensity and structure forecasts in HWRF, Mon. Wea. Rev., 143, 3136-3155
2014
Dunion, J.P., C.D. Thorncroft, and C.S. Velden, 2014: The tropical cyclone diurnal cycle of mature hurricanes. Mon. Wea. Rev., 142, 3900-3919, https://doi.org/10.1175/MWR-D-13-00191.1.
Looking for Literature? Search our Publication Database.